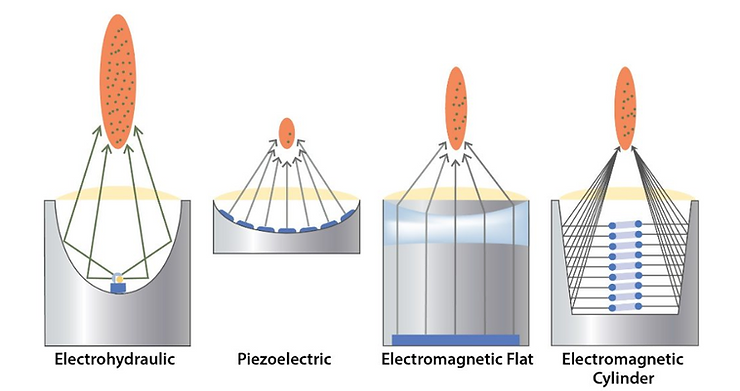
Exploring the Science of Shock Waves in Therapeutic Applications
Shock waves, characterized by abrupt and nearly instantaneous changes in pressure, play a unique role in various therapeutic applications, from lithotripsy to musculoskeletal treatments. These waves travel faster than sound, allowing them to transmit mechanical energy deep into tissues, stimulating healing and repair. This article delves into the physical principles of shock waves, the types of shock wave generators, and their application in medical settings.
The Physics of Shock Waves
In physical terms, a shock wave is a powerful pulse with a broad frequency range (typically from 150 kHz up to 100 MHz), high pressure amplitudes (up to 150 MPa), and low tensile amplitudes (up to -25 MPa). Shock waves also feature an extremely short rise time of just a few hundred nanoseconds and a narrow pulse width, producing a steep, almost vertical pressure profile. When used therapeutically, focused shock waves penetrate tissue layers to stimulate biological responses, activating cellular repair processes in a controlled, non-invasive manner.
Types of Shock Wave Generators
There are three main types of shock wave generators: electrohydraulic, electromagnetic, and piezoelectric. Each employs a different method to produce shock waves with specific characteristics and therapeutic uses.
Electrohydraulic Generators Electrohydraulic shock waves are produced through a spark discharge in water. This type of generator uses a high-voltage electrode placed at the focal point within a water-filled semi-ellipsoid reflector. When an electric current is applied, a spark creates a plasma bubble that rapidly expands, releasing a spherical shock wave. This wave spreads, creating an initial radial wave followed by a focused shock wave through the reflector’s curved surface.
Electromagnetic Generators Electromagnetic generators create shock waves through the use of a coil and metal membrane. When the coil is charged, it propels the metal membrane away, generating a low-pressure acoustic pulse. There are two variations of this design:
Plane Wave Creation: Uses an acoustic lens to focus the generated plane wave to a specified focal point.
Cylindrical Source: Produces a cylindrical wave that is focused by a hyperbolic metal reflector, forming a focused shock wave.
Piezoelectric Generators This method harnesses the piezoelectric effect, where certain materials generate sound waves when an electric field is applied. In piezoelectric shock wave devices, hundreds of piezoelectric crystals are arranged on a spherical surface. When energized, the crystals create a low-pressure pulse that self-focuses due to the spherical arrangement, eliminating the need for lenses or additional focusing mechanisms. Double layers of piezo elements are sometimes used to increase pulse energy, making these generators precise and effective for targeted treatments.
Focused vs. Radial Shock Waves
While focused shock waves penetrate deep into tissues, radial shock waves are generally confined to superficial tissues and behave more like pressure waves. Radial shock wave devices generate acoustic pulses through the ballistic principle, where a projectile is accelerated by air pressure or electromagnetic forces, hitting an applicator to produce a wave. The highest energy of radial shock waves is concentrated at the applicator’s surface, making them suitable for treating superficial conditions rather than deep tissue.
Challenges in Shock Wave Therapy: Reflection, Refraction, and Absorption
As shock waves travel through various tissue types, their amplitude and sound field can be influenced by reflection, refraction, diffraction, and absorption. For example, reflection at air-tissue interfaces (such as air pockets or gas bubbles) can reduce the wave’s energy by up to 99%, significantly impacting therapeutic efficacy. Coupling agents like ultrasound gel are essential to minimize these effects and ensure consistent energy transmission.
In medical applications, shock wave generators are typically used with a coupling medium that eliminates air gaps between the transducer and the patient’s skin, optimizing the delivery of therapeutic energy. Care must be taken in treating areas near air-filled organs like the lungs or intestines, as the reversed pressures from wave reflection at air interfaces could potentially harm these tissues.
Measuring Shock Wave Parameters
Standards like IEC 61846 outline specific shock wave parameters that describe focused shock wave fields, including:
Energy Density: The concentration of energy delivered per unit area.
Maximum and Minimum Pressure: Peak and trough pressures.
Spatial Expansion: The focal area’s dimensions, defining how much tissue area the wave impacts.
These parameters are typically measured with a fiber optic probe hydrophone in a controlled water tank to ensure consistency and accuracy. However, when applied to human tissue, these parameters can change due to the variable nature of biological tissues, which may alter the wave’s intensity and distribution.
Advanced Therapeutic Applications
Today, shock wave therapy is a trusted method for treating kidney stones, chronic tendinopathies, and musculoskeletal pain. Devices using electrohydraulic, electromagnetic, or piezoelectric technologies allow clinicians to deliver customized treatments for various tissue depths and conditions. The selection of generator type depends on the condition being treated, the depth of tissue penetration required, and the precision needed.
Conclusion
Shock wave therapy harnesses powerful acoustic pulses to promote healing and regeneration, taking advantage of unique physical properties to deliver energy deep into tissues. With continuous advancements in generator technology, from electrohydraulic to piezoelectric systems, shock wave therapy remains a versatile, non-invasive solution in modern medicine. By understanding the physics of shock waves and refining generator technology, researchers and clinicians continue to expand shock wave therapy’s therapeutic potential across numerous medical fields.